This is the story of the revolutionary development of mini-organs (organoids) and their countless applications on how they help us to develop and implement tailored therapy for each patient. A tale for the future of medicine from a group of scientists in Utrecht that started it all - out of wonder.
Me and my organoid: a step closer to tailored therapy
Estimated reading time: 16 minutes

No one wants to die of cancer, inherit a congenital disease or witness the daily pain and agony of our loved ones, ravaged by diseases tough to beat. Yet, on the day you read this, over 3,500 people will die of cancer in the EU alone, and about 5,000 people will be diagnosed with it. Twenty-two others - from Uruguay to France to New Zealand - will die awaiting an organ that could have saved their lives. And then there are the 152 million people who, by 2050, are expected to suffer from Alzheimer's or other dementias.
As we age and live longer, more people will become ill. How do we deal with the struggle to survive an illness?
No two people are alike…
Every person has their own construction plan encoded inside the DNA. And although it’s said that a meagre 0.1% of DNA is what makes us truly different, millions of mutations and variations can happen anywhere in our genome any given day. These small variances in our genetic make-up, alongside our age, environment and lifestyle choices, make a huge difference in how any two people metabolise caffeine or react to a drug or treatment. The result: no two patients are alike.
And yet, medicine remains highly impersonal.
Despite improving the lives of millions over the past few decades, its success has relied on averaging standard treatments. In her 10 years as a medical oncologist at the University Medical Center Utrecht, Miriam Koopman, has seen enough. She felt impotent by having to administer adjuvant chemotherapy to her patients with early stage colon cancer (stage III) knowing that it would "only work for two out of ten." The cancer would come back after treatment to three of those patients, and the other five could have done without chemotherapy (and its side-effects). And yet, this is the best cancer treatment we have got to improve their chances of recovery.
I would like to know in advance which patients will benefit from which cancer treatment.
Things can be done differently… What if you could know beforehand which treatment works for your specific situation?
In 2009, Hans Clevers, a stem cell biologist at Utrecht University, made a discovery that would forever transform healthcare. His lab at the Hubrecht Institute had grown what, under the microscope, looked like a real gut outside the body (made from mouse intestinal cells). It was the first in a series of organs-in-a-dish that would revolutionise personalised medicine in every corner of the world.
Today, the possibilities of these mini-organs are enormous: from finding the right treatment to otherwise lethal genetic diseases, numerous cancers and infectious diseases, to understanding furtive neurological disorders such as schizophrenia, bipolar disorder or autism without having to poke one’s brain. We also know deaths from organ donor shortages could soon be a thing of the past.
Welcome the organoids: mini-organs that could save your or your family’s life one day…
But how do organoids work exactly? What can you do with organoids now, and which doors will they open for patients and healthcare in the future? They may sound like science fiction to you, but organoids are already transforming patients’ reality.
What are organoids?
The thought of mini-brains in a dish may reverberate the horrors of Ridley Scott’s film Blade Runner, or the much older black-and-white film Donovan’s Brain. The term itself has a science fiction sound to it. The reality however is much less histrionic, with ‘organoid’ used to describe 3D structures of human or animal tissue grown from stem cells. Simply put, organoids are miniaturised and simplified versions of organs grown outside the body. They are tiny, just a millimeter or less in diameter, far from the size and shape of, say, a liver or a brain. Although they are not yet like real organs – they lack the structure and interconnectivity of actual human organs, such as the blood vessels that provide access to the circulatory system - they mimic them quite authentically. Organoids are revolutionising science and healthcare, pushing the envelope of personalizing medical treatments.

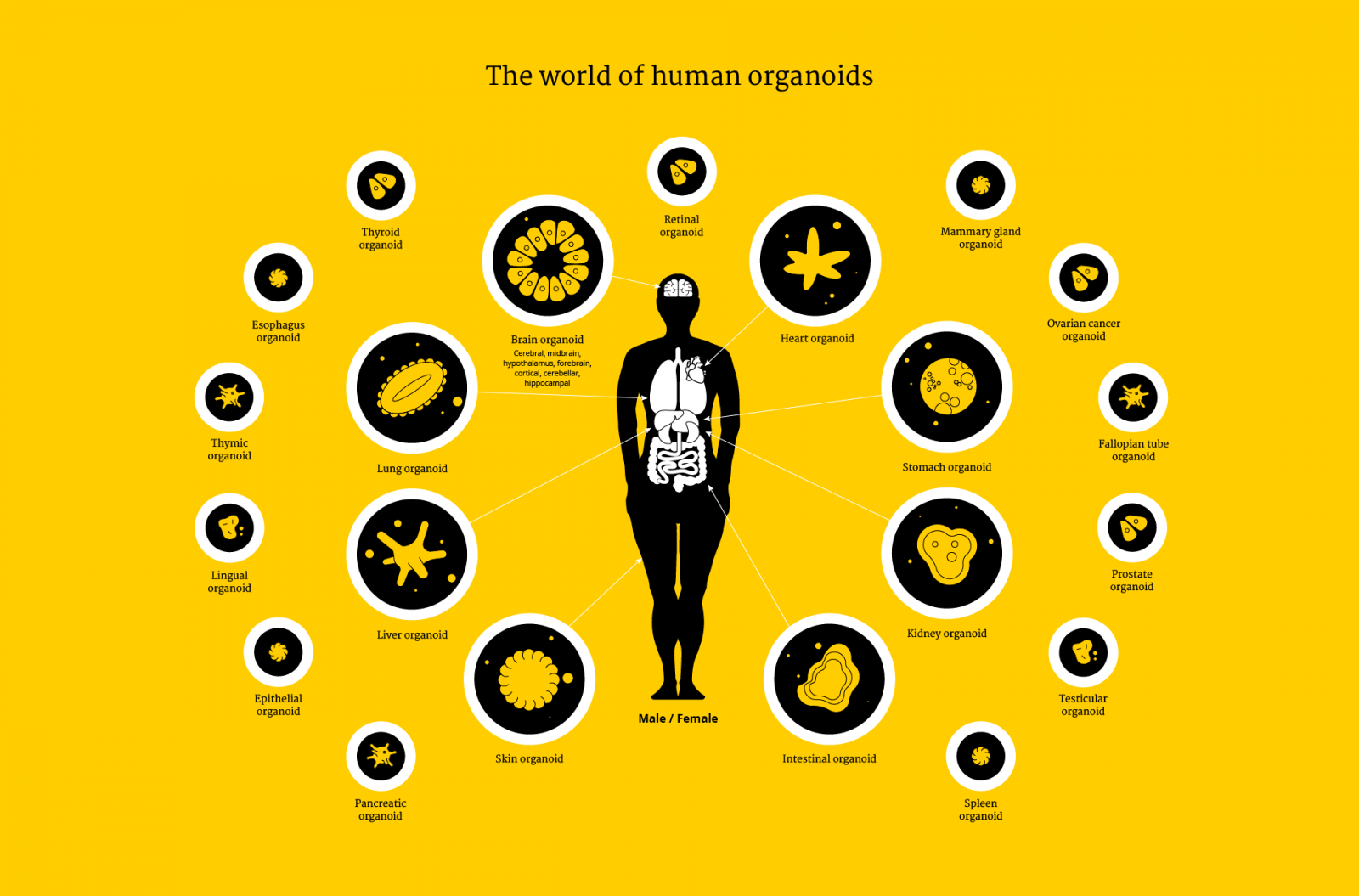
Over the last decade, researchers at Utrecht University have made organoids from almost every living tissue: liver, kidney, intestine, lung, retina, tongue, heart, pancreas and the brain. Stem cells are the main ‘ingredient’ for organoids, but not the only one – growing organoids that recapitulate the micro-anatomy of a real tissue or organ faithfully requires getting the recipe just right for each of them. A fascinating thing in the process of ‘culturing’ or growing organoids is that stem cells divide and self-assemble into recognizable tissues without the input from an entire body. One example of this is bundles of brain neurons that coax together to transmit nerve impulses in a brain organoid.
As organoids are similar to original organs, scientists use them to study organ development and diseases. These mini-organs can be stored in biobanks and can be used for scientific research, drug screening, disease modeling, precision medicine and regenerative medicine.
The exciting part is that they can be personalized. One day, scientists may be able to routinely take your stem cells (from a small sample of tissue or biopsy), bath them in a chemical cocktail and let them self-assemble and grow into a miniature version of your liver or your kidney in a dish. All this in order to test the efficacy of a drug and see how you will respond to a medication – a giant leap in personalised medicine the size of a chia seed!
What can we do with organoids?
The universe of organoids keeps increasing, and so does the range of research and clinical applications. Here are some of the most promising results achieved in Utrecht, from finding a cure for patients who would otherwise be excluded from treatment, to reducing the costs of healthcare and animal experimentation, to becoming an alternative to organ donation.

Follow your mini-gut’, it can be the right medicine for you
We are often told to trust our gut feeling when it kicks in – it’s our second brain after all. Incidentally, mini-guts have indeed demonstrated to be a reliable tool for determining in advance whether or not a drug will work on individual patients.
This discovery has been built upon the gut organoids developed in Hans Clevers' lab. A research team led by molecular biologist Jeffrey Beekman at UMC Utrecht generated mini-intestines from patients with cystic fibrosis to test whether they could predict the effectiveness of existing drugs. It’s worth noting that these drugs cost around 200,000 euros per patient per year, while only a small proportion of patients with common mutations are reimbursed for them. What if there was proof that a drug works for a patient with a rare mutation? The study found that the organoids could tell with high accuracy which patients would respond to the therapies. In just a few weeks and at a cost of less than 3,000 euros per patient alone, a process that normally takes years by directly trialing the therapies on patients themselves.
In 10 years from now we can solve the problem of cystic fibrosis and make drugs available for over 99% of patients.
Beekman’s research results were so resounding that a positive organoid test now offers a chance to people with cystic fibrosis in The Netherlands to receive medication that would otherwise never be available to them.
Millimetric organoids are making a big difference in people’s lives. At the start of 2017, UMC Utrecht and the Dutch Cystic Fibrosis Foundation (NCFS) signed an agreement with the ambition of extending individualised treatment for the more than 1,500 people who live with cystic fibrosis in The Netherlands. Fast forward to 2020, and this successful drug screening method on organoids is extended to over 500 European patients of cystic fibrosis with rare genetic profiles to test on which mutations candidate drugs have a positive effect.
Personalised medicine for cystic fibrosis: a timeline
A future alternative to organ transplants
One of the major risks of organ transplants is our body’s rejection. It makes sense - if we take cells from another person’s body and put them into our own, white blood cells see them as foreign and try to attack them. The exciting part about patients-derived organoids is that they carry an individual’s own cells, so using organoids in transplants could avoid the common risk of tissue rejection.
These organoids’ caveat is that they can’t possibly recapitulate complex functions as a real organ does in the body. Bart Spee, associate professor at Utrecht University’s Faculty of Veterinary Medicine, wanted to find out whether transplanting healthy stem cells into the livers of dogs would help repair their damaged organ completely. The idea was promising – the researchers hoped that those 700,000 healthy stem cells could replicate in vivo and ultimately win over the bad cells. The transplanted cells did indeed survive in the dogs’ livers, but unfortunately, didn’t replicate and regenerate these dogs’ livers.
As an alternative approach to cell transplantations, Bart Spee and his colleague Kerstin Schneeberger are currently combining biofabrication technologies with organoids to create liver tissue in the lab, which can then be transplanted into a damaged patient liver. They are bringing their experience to a new consortium, OrganTrans, led by the Swiss research and technology organization CSEM, to develop a bioprinter that can print pieces of liver constructs from the cells of patients. “Making an entire liver is not easy,” Spee stresses, "but this unique collaboration can go a long way, as it includes the entire process of stem cell production, bioprinting, control systems and functional testing of the liver constructs." "We hope that these 3D bioprinted livers can become a solution for patients with liver diseases and for the fatal donor organ shortage worldwide," adds Kerstin Schneeberger.
My goal is to transplant a functional bioprinted liver construct into a patient.
Saving lives, saving money
Drug research and development is expensive and time consuming, which is particularly frustrating for somebody with a life-threatening or life-impairing disease waiting for a new treatment. It takes approximately 10 years to move a new drug from research lab to patient, at a median cost of around 1 billion euros.
Why so expensive? In part, because the overall costs factor in all the failed attempts that preceded it. And in pharmaceutical research and development, there are many failed attempts. Only 1 in 5,000 drugs reach the market. Many drop in preclinical trials on animal models, and an underwhelming 15% of those compounds passing onto human clinical trials are approved for commercialisation.
Human clinical trials are an expensive component of the drug discovery pathway, which often fail due to the fundamental differences between humans and animal model physiology.
But now with organoids, scientists can screen multiple therapies at once, streamlining that process and helping to reduce the number of animals used for research. New compounds or combinations of drugs can be tested on human organoids earlier, discarding unsafe, ineffective or toxic drugs immediately. In turn this helps speed up new drug discoveries and reduce the overall costs of medicines.
Many drugs get pulled from the market because they are toxic to the liver. Miniature livers can be a great proxy for early drug-toxicity testing.
Mini organs, big dreams
The applications of organoids are limitless. Organoids can be grown for almost any organ, to test a battery of drugs for potentially any disease before prescribing the right treatment to an ill person. Researchers in Utrecht are growing replicas of cancer patients’ tumors to find therapies that work best for their specific mutations. They also infect organoids with parasites in order to better understand host-microbe interactions that can help outsmart bacteria. And the researchers develop novel vaccines or therapeutic approaches to widespread diseases such as diabetes.
We’re getting closer to being able to design therapy for each patient’s unique case of cancer.
With time, we might eventually even untangle the mysteries of the brain and treat a host of neurological disorders such as schizophrenia or neurodevelopmental disorders such as autism and ADHD. Neuroscientist and Chair of the UMC Utrecht Brain Center Jeroen Pasterkamp is already using brain organoids to study the first 1001 days of our existence, which is a crucial period for brain development that can give clues in understanding what causes learning development disorders.
With the help of organoids we can understand developmental diseases of the brain better and, in time, also treat them better.
If you think mini-brains aren’t cool enough, look at the most recent tweak to the organoid technique – to grow snake venom gland organoids, which can become a rich source of anti-venom and other medicines.
It takes a village to nurture organoids
Brain organoids, for example, can be grown from blood cells or skin cells extracted from your body and cultured in a cocktail of nutrients that induce them to differentiate and become brain tissues. Intestinal stem cells can be isolated from a small tissue sample removed from a patient with a biopsy or other means to then self-assemble into a mini-gut. Whatever the method, it has become easier with time and cross-sharing. But growing organoids still takes lots of specialists, logistics and infrastructure.
All the human tissue required for organoids needs to be kept alive immediately after being removed from a patient’s body so that it is of use to researchers. The Utrecht Platform for Organoids Technology (UPORT) was founded to coordinate a whole host of medical specialists, from surgeons to nurses to pathologists to radiologists to oncologists to researchers. Including patients themselves, who need to give their consent for the use and storage of their bodily material in an organoid biobank before entering the operating room.
“Together, we’ve designed and implemented a pipeline for tissue acquisition and processing,” explains Anneta Brousali, study coordinator at UPORT. Anneta acts on behalf of different research teams, ensuring patients’ informed consent, and liaises between the surgeon and the pathologist. “Aside from the fascination of helping to dissect tumor biopsies, I’m there to make sure we get enough tissue for the particular research project,” she explains.
With the Utrecht Platform for Organoid Technology researchers and doctors have a stable framework to offer patients tailor-made treatments.
How to develop ethically sound organoids?
Just a tiny piece of tissue from a surgical intervention or blood test is necessary for scientists to grow indefinite mini-guts, mini-brains or many other body parts. Next, they can then be stored in biobanks for long periods of time and picked up later to decode the genetic complexity of cancer, fight against infections or discover an effective new treatment.
Exciting, of course, but it also brings up ethical questions…
Those balls of tissue frozen and preserved at a temperature of -80 degrees Celsius contain an individuals’ genotype and phenotype (DNA, identity, values…); all privacy-sensitive information encapsulated in your cells.
Body parts, tissues and fluids are removed from patients every single day. While much of that bio-material is willingly donated to science or medicine, some of that tissue has been obtained in the past in a ‘catch what you can’ fashion at operating rooms. Given the extraordinary nature of organoids, how do we ensure the tissue needed for organoids is acquired in an ethically appropriate manner?
As the birthplace of organoids, Utrecht University brought together the science, technology and ethics of organoids. Professor of Bioethics Annelien Bredenoord, together with Hans Clevers, Edward Nieuwenhuis, Kors van der Ent and others launched an initial project to proactively examine the ethics of organoid technology, including ethical questions concerning the derivation, storage and use of organoids.
These novel dilemmas also raised professional responsibilities with the setup of Hubrecht Organoid Technology, a foundation established with the organoid patents of the Hubrecht Institute, in order to support the pharmaceutical industry implement new technologies for drug discovery.
One of the challenges for organoid biobanks is to maintain the privacy of account holders, so Hubrecht Organoid Technology codes each specimen so that the tissue sample remains unidentifiable. "A patient's medical data are never shared. Only with specific consent and then only anonymized," emphasises Robert Vries, CEO of Hubrecht Organoid Technology.
HUB Organoids are so closely related to the patient that we can use them to predict a patient's response to drug treatment.
Bredenoord and colleagues also conducted patient participation studies and developed a governance framework for organoid biobanking. The unpredictable development of organoids, together with the value patients adhere to them, requires a paradigm of ongoing governing obligations from biobanks that transcend the limits of initial consent and include continuous ethical oversight, participant engagement, benefit sharing and privacy by design.
At the Hubrecht Organoid Technology, every time researchers want to use a sample from the biobank, it requires approval from the ethical board for biobanking studies, explains Robert Vries. The ethics board makes sure the use of donor tissue complies with the permissions given by the patient through the consent form, Annelien Bredenoord adds.
“We set up a programme in which we involved patients to think with us about fair use, fair licensing and pricing.”
There is healthcare today because the generations before us were willing to join clinical trials, and altruistically donate their tissues for use in research. We, as future patients – and we are all future patients, also have a moral duty with the generations after us.
What’s next? The future of personalized medicine
An exciting future vision is one where organoids enter every doctor’s office so that personalised medicine reaches everyone without exception. Imagine the day that you can walk into your GP’s room abated with a disease, get a DNA test done within seconds, and walk out with a tailored therapy guaranteed to kill those bad cells based on your organoid’s response.
Making organoids a reality for every patient in the future requires investment now to scale up the technology.
Growing mini-organs or mini-tumors, testing and analysing them is a time-consuming process. We’ll need robots to mass-produce mini-organs in a fraction of the time it takes scientists now. Also we’ll need the integration of artificial intelligence and bioinformatics to screen phenotypes and genotypes to search for the right drug to the right patient at the click of a button. Most importantly, we need clear guidelines and trained professionals to ensure tissues are obtained in an optimised and standardised manner, in compliance with all relevant regulations. And we need support – from governments to health insurers to citizens, and everyone in between to take a leap forward for a better health and a better future.
In the meantime, if you are planning to undergo a surgical intervention soon, check out the option to donate your tissue…
My dream is that anybody with a diseased organ can pick an organoid from the biobank and receive a transplant.